A Scanning Electron Microscope (SEM) magnifies images by using electrons instead of light. This technology allows for high-resolution observation.
The Scanning Electron Microscope (SEM) is a pivotal tool in modern scientific research. It enables experts to view objects at magnifications up to 2,000,000 times. Unlike traditional light microscopes, SEMs employ a focused beam of high-energy electrons to produce a variety of signals at the surface of solid specimens.
These signals reveal information about the sample, including texture, chemical composition, and crystalline structure, offering a detailed look at a microscale world otherwise invisible to the naked eye. With its unique capabilities, SEM has become indispensable across various fields, from material science and biology to forensic engineering and nanotechnology. Users appreciate the depth of focus and three-dimensional imaging that provide insights into the topography and morphology of examined samples. The technological advancements in SEM have continually pushed the boundaries of discovery, rendering it a cornerstone of observational science.
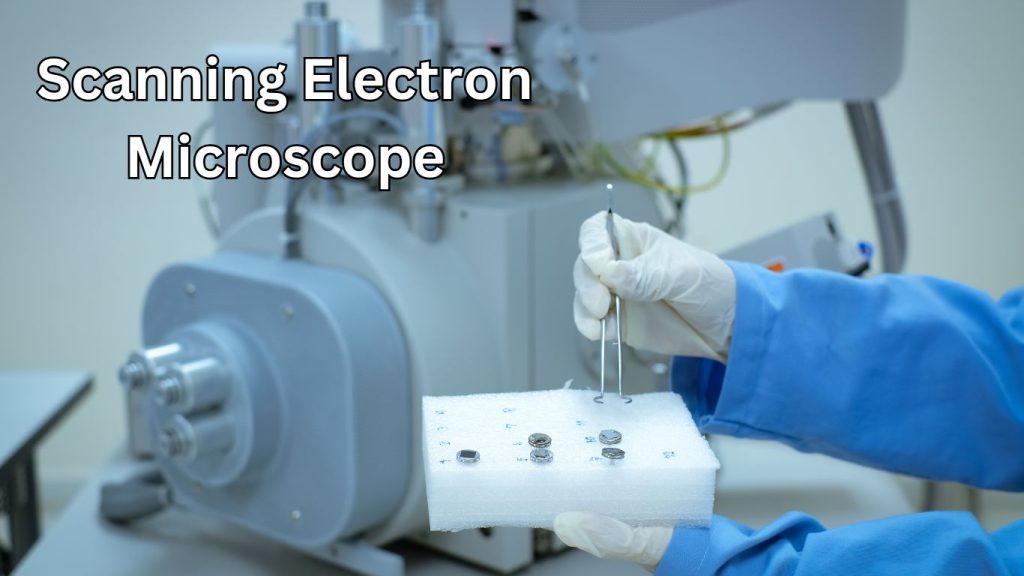
Learn more about Simple Microscope in the main guide.
Introduction To Scanning Electron Microscopy
Embark on an intriguing journey into the miniature world with the Scanning Electron Microscope (SEM). This powerful tool has revolutionized how we observe the tiniest details that elude the capabilities of traditional light microscopes. Whether for advanced research or practical applications in material science, biology, and beyond, the introduction to Scanning Electron Microscopy is a gateway to a world of nanoscale exploration.
Overview Of Electron Microscopy
Electron microscopy is at the forefront of imaging technology, offering magnifications that delve deep into the cellular level and beyond. Unlike optical microscopes, which use light to illuminate samples, electron microscopes employ a focused beam of electrons. This results in higher-resolution images where even atoms can be discerned. Within this category, Scanning Electron Microscopy (SEM) emerges as a titan of surface imaging, revealing textures, structures, and compositions in astonishing detail.
Principles Of Sem Operation
- Electron Generation: Within the SEM, an electron gun emits high-energy electrons directed towards the sample.
- Electron Focusing: A series of electromagnetic lenses focus the electrons into a narrow beam.
- Scanning Process: The beam then sweeps across the sample’s surface in a rasterized pattern.
- Interaction with Sample: As electrons interact with the sample, they generate secondary signals such as secondary electrons, backscattered electrons, and X-rays.
- Signal Collection: Detectors capture these emitted signals to form a detailed image that reflects the sample’s surface topography and composition.
Components Of A Sem
Component | Function |
---|---|
Electron Gun | Generates the primary electron beam. |
Electromagnetic Lenses | Condense the electron beam to ensure precision scanning. |
Sample Chamber | Hosts the specimen under vacuum conditions. |
Detectors | Capture various types of signals produced by electron-sample interactions. |
Display System | Converts the signals to a visual image for analysis. |
Computer System | Processes the data and controls the SEM’s functionality. |
Applications And Advantages Of Sem
Exploring the microcosm has never been as detailed and accessible as it is with the impressive capabilities of the Scanning Electron Microscope (SEM). A mainstay in modern research facilities and laboratories, SEMs utilize focused beams of electrons to provide high-resolution images of the surfaces of materials. This powerful tool goes beyond mere imaging; it aids in elemental analysis and offers revealing insights into the composition of biological, chemical, and physical samples. Let’s dive into SEM’s various applications and inherent advantages in different fields.
Material Science And Engineering
The field of material science and engineering benefits greatly from SEM by unearthing microstructural details that are imperative for understanding and improving material properties. Applications include:
- Characterization of Nanomaterials: Assessing size, shape, and distribution.
- Analysis of Fractures: Identifying failure modes in metals and polymers.
- Surface Coatings: Examining thickness, uniformity, and adhesion.
Biological And Medical Research
In biological and medical research, SEM offers unparalleled views into the building blocks of life. Researchers leverage SEM to:
- Examine Cell Morphology: Understanding the structure of cells and tissues.
- Analyze Microorganisms: Studying bacteria and viruses in high detail.
- Investigate Pathological Changes: Discovering alterations at the disease site.
Semiconductors And Electronics
With the ever-shrinking scales in the semiconductors and electronics industry, SEM becomes integral for validating manufacturing processes and ensuring quality control. Key uses include:
- Inspection of Circuitry: Detecting defects and verifying nanoscale dimensions.
- Material Purity Analysis: Ensuring that materials meet stringent industry standards.
- Development of Microelectromechanical Systems (MEMS): Enabling innovation in miniaturized devices.
Advantages Over Other Microscopy Techniques
SEM offers several advantages over other microscopy techniques, setting it apart as a highly esteemed tool in research and industry:
SEM Advantage | Description |
---|---|
Higher Resolution: | Ability to view objects down to a few nanometers. |
Greater Depth of Field: | Produces clearer 3D images for analyzing surface topography. |
Versatile Sample Handling: | Can accommodate a wide range of sample types, sizes, and conditions. |
Compositional Analysis: | Can detect and quantify the presence of various elements in the sample. |
Procedure And Sample Preparation
Scanning Electron Microscopy (SEM) has revolutionized how we observe the microscopic world, offering magnification levels that reveal intricacies down to the nanometer scale. A critical component of achieving such vivid images lies within the Procedure and Sample Preparation. Preparing a sample for SEM analysis demands precision and an understanding of the unique sample characteristics to avoid damage and enhance image quality. Let’s dive into the meticulous world of SEM sample preparation and imaging process.
Sample Preparation Techniques
Sample Preparation Techniques
The integrity of SEM imaging hinges upon the sample preparation techniques. A well-prepared sample ensures high-quality images and a wealth of information. Preparation involves a series of steps meticulously tailored to the specimen’s properties and the desired outcome.
- Drying: Samples must be completely dry to prevent damage under the vacuum conditions in the SEM chamber.
- Mounting: Specimens are affixed to SEM stubs using conductive adhesive tapes or paints, ensuring they remain static during imaging.
- Coating: Non-conductive materials often require a fine conductive coating to prevent charging and improve image clarity.
- Sectioning: Biological specimens are sometimes embedded in resin and sectioned for detailed cross-sectional analysis.
Key Steps in the SEM Imaging Process
Key Steps In The Sem Imaging Process
The SEM imaging process is a sequence of detailed steps that takes place within the electron microscope’s vacuum chamber. This process unveils the fine details of a specimen’s surface topology and composition.
- Loading: Samples are inserted into the SEM chamber and secured.
- Vacuum: The chamber is evacuated to create the necessary vacuum for electron beam stability.
- Alignment: An electron beam is aligned with the sample’s surface.
- Scanning: The electron beam systematically scans the surface area.
- Detectors: Secondary or backscattered electrons are detected, transformed into a signal, and displayed as images.
Dealing with Non-conductive Materials
Dealing With Non-conductive Materials
Non-conductive materials present a unique challenge in SEM, as they are prone to charge buildup, which can distort the image. To mitigate this, a thin layer of conductive material, such as gold, platinum, or carbon, is often sputter-coated onto the sample’s surface. This creates a path for the electrons to the ground, ensuring a clear, charge-free image. Critical point drying is another technique employed to prepare delicate specimens without damaging their structure, preserving their morphology for accurate observation.
- Sputter Coating: A method to apply a thin conductive film onto the specimen.
- Carbon Coating: An alternative to metal coatings, useful for specific analytical tasks.
- Critical Point Drying: Drying sensitive biological samples without surface tension effects.
Advanced Sem Techniques
Welcome to the world of Advanced Scanning Electron Microscope (SEM) Techniques, where the microscopic realm is rendered visible and dynamically analyzed to unveil the secrets of a material’s composition and properties. These cutting-edge SEM technologies have propelled scientific research and material science into an exciting new era. Let’s delve into the specific techniques that represent the pinnacle of SEM technology.
Energy-dispersive X-ray Spectroscopy (eds)
Energy-dispersive X-ray Spectroscopy, commonly called EDS or XEDS, is a hallmark of modern SEM analysis. This technique utilizes the principle that each element has a unique atomic structure, allowing it to emit a unique set of X-rays, which EDS then detects and measures. These measurements enable precise elemental analysis of the sample. Notable applications include:
- Material characterization: Determining a sample’s elemental composition helps identify and confirm the presence of specific elements within a material.
- Defect analysis: By mapping the elemental distribution, EDS can reveal inconsistencies and impurities affecting material performance.
- Qualitative and quantitative analysis: Provides both the types of elements and their relative abundance within the sample.
Environmental Sem (seem)
Unlike traditional SEM, which requires a high vacuum environment, the Environmental Scanning Electron Microscope (ESEM) allows for observing samples in a gaseous atmosphere. This unique feature transforms the capabilities of SEM in several ways:
ESEM maintains a hydrated environment for the sample, which is essential for examining biological specimens, wet materials, or any sample that would be damaged under high-vacuum conditions. The ability to control moisture levels also opens up possibilities for studying dynamic processes, such as:
- Corrosion: Monitoring how materials degrade or corrode in real-time.
- Drying processes: Observing how a material’s structure changes as it loses moisture.
- Chemical reactions: Investigating reactions in specific environmental conditions.
Focused Ion Beam (fib) Sem
Integrating precision material ablation with imaging, focusing ion beam (FIB), and SEM is a powerhouse technique for material scientists and engineers. FIB utilizes a beam of ions, typically gallium ions, to mill the surface of a sample with nanometer precision. This milling ability, combined with SEM imaging, allows for a plethora of applications:
Application | Description |
---|---|
Site-specific analysis | Allows targeted investigation of specific regions within a sample, making it invaluable for examining integrated circuits and nanostructures. |
3D reconstruction | By sequentially milling and imaging, FIB can compile a series of cross-sectional images to recreate a three-dimensional model of the sample. |
Sample preparation | Enables the creation of thin sections of samples suitable for Transmission Electron Microscopy (TEM) and other high-resolution techniques. |
Understanding Sem Images
The Scanning Electron Microscope (SEM) is a powerful tool that provides detailed images of a material’s surface. To truly benefit from these high-resolution images, one must understand the intricacies behind their interpretation. This section delves into the process of interpreting SEM images, recognizing common artifacts, and utilizing quantitative analysis and image processing techniques to extract reliable data.
Interpreting Electron Images
When examining SEM images, we must recognize that we observe a complex interplay of electrons interacting with the sample. Here’s how to decipher these visuals:
- Contrast: Variations in the image’s brightness often indicate differences in the material’s composition or surface topography.
- Scale: SEM images come with a scale bar; understanding the size of the features you’re looking at is crucial for accurate interpretation.
- Depth: The shadowing effects in the image can help ascertain the 3-dimensional structure of the surface.
Common Artifacts In Sem
Artifacts are common in SEM images and can be misleading. Recognizing these allows for a more accurate assessment of the data:
- Charging: Non-conductive materials can accumulate charge, leading to bright spots or areas in the image.
- Edge Effect: Edges and corners can appear especially bright due to an increased number of emitted electrons.
- Contamination: Any foreign material can cause spurious signals that distort the true nature of the sample’s surface.
Quantitative Analysis And Image Processing
Technology advancements have enhanced SEM capabilities, allowing for detailed quantitative analysis and sophisticated image processing. Here are some ways these techniques support SEM image interpretation:
Feature Measurement | Image Segmentation | Texture Analysis |
---|---|---|
Size: Accurately measure the dimensions of the sample’s features. | Phase Identification: Separate different phases or components within the image. | Surface Roughness: Determine the texture characteristics of a surface. |
Distribution: Analyze the spatial distribution of elements or compounds. | Thresholding: Convert grayscale images into binary for easier interpretation. | Pattern Recognition: Identify repeating structures or defects. |
Challenges And Future Of Sem
The Scanning Electron Microscope (SEM) is a monumental instrument in material science, biology, and beyond. It offers an unparalleled view into the microcosms that compose our world, yet it does not come without its challenges. As we continue to push the frontiers of science and technology, SEM must evolve to meet the dynamic needs of modern research. The quest for highly detailed and reliable imagery prompts ongoing innovation, nurturing a vibrant future for SEM in scientific discovery.
Limitations Of Current Sem Technology
One of the key factors that impede current SEM technology is its intrinsic limitations in resolution, depth of field, and sample preparation:
- Resolution Constraints: While SEMs offer magnification far beyond traditional light microscopes, they still face an upper limit imposed by factors such as electron beam interactions and lens aberrations.
- Sample Damage: High-energy electron beams can alter or damage sensitive samples, challenging certain types of analysis.
- Conductivity Requirements: Conductive specimens are ideal for SEM analysis, requiring non-conductive materials to be coated, possibly affecting the true nature of the sample.
- Vacuum Environment Necessity: SEM typically operates in a vacuum, precluding the direct observation of living or wet specimens without specialized preparation.
Emerging Innovations In Sem
Researchers and technologists have been diligently working to transcend these barriers, leading to groundbreaking innovations in SEM:
- Improved Detectors: Advanced detection technology enhances image quality and resolution while reducing sample damage.
- Low-Voltage SEM: Development in low-voltage electron microscopy minimizes damage to delicate samples and improves surface detail imaging.
- Environmental SEM: ESEM technologies enable the observation of specimens in their natural state, including wet and insulating materials.
- 3D Reconstruction: Combining SEM with computational methods allows for intricate three-dimensional reconstructions of microstructures.
The Role Of Sem In Future Scientific Research
The role of SEM in future scientific endeavors will largely depend on its capacity to adapt and improve. Scientists anticipate:
Advancements | Impact |
---|---|
Automation and Artificial Intelligence | Efficiency in specimen analysis and intelligent pattern recognition. |
High-Throughput SEM | Faster data acquisition, enabling larger scale studies. |
In-Situ Capabilities | Real-time observation of dynamic processes in materials science and biology. |
Correlative Microscopy | Integrated techniques that expand the capacity for multimodal analysis of samples. |
SEM is boldly leading us toward a future of discovery. It will continue to unveil the intricacies of our universe, one electron at a time. Google Maps.
Frequently Asked Questions On Scanning Electron Microscope
What Is A Scanning Electron Microscope Used For?
A scanning electron microscope (SEM) views high-resolution, three-dimensional images of microscopic structures and surfaces. It analyzes surface topography and composition by scanning samples with a focused electron beam.
What Is the Difference Between Sem And Tem?
SEM (Scanning Electron Microscopy) and TEM (Transmission Electron Microscopy) differ in imaging techniques. SEM scans a sample’s surface to create an image, while TEM transmits electrons through a specimen for internal structure analysis. Each offers unique microscopic insights.
What Is The Sem Analysis Used For?
SEM analysis helps identify elements of a material’s composition and characterizes its chemical properties.
What Is Sem Used To View?
SEM, or Scanning Electron Microscopy, is used to view the surface topography and composition of materials on a microscopic scale.
Conclusion
Exploring the microcosmos has never been as insightful as with a scanning electron microscope (SEM). This advanced tool unveils the minutiae of our world with astounding clarity. SEMs are pivotal in pushing the boundaries of science and technology, whether for research or industry.
As we embrace these powerful instruments, we continue our quest to unveil the invisible, one electron beam at a time.